animal models
The Hidden Beauty of Intestinal Villi
Posted on by Dr. Francis Collins

The human small intestine, though modest in diameter and folded compactly to fit into the abdomen, is anything but small. It measures on average about 20 feet from end to end and plays a big role in the gastrointestinal tract, breaking down food and drink from the stomach to absorb the water and nutrients.
Also anything but small is the total surface area of the organ’s inner lining, where millions of U-shaped folds in the mucosal tissue triple the available space to absorb the water and nutrients that keep our bodies nourished. If these folds, packed with finger-like absorptive cells called villi, were flattened out, they would be the size of a tennis court!
That’s what makes this this microscopic image so interesting. It shows in cross section the symmetrical pattern of the villi (its cells outlined by yellow) that pack these folds. Each cell’s nucleus contains DNA (teal), and the villi themselves are fringed by thousands of tiny bristles, called microvilli (magenta), which are too small to see individually here. Collectively, microvilli make up an absorptive surface, called the brush border, where digested nutrients in the fluid passing through the intestine can enter cells via transport channels.
Amy Engevik, a researcher at the Medical University of South Carolina, Charleston, took this snapshot to show what a healthy intestinal cellular landscape looks like in a young mouse. The Engevik lab studies the dynamic movement of ions, water, and proteins in the intestine—a process that goes wrong in humans born with a rare disorder called microvillus inclusion disease (MVID).
MVID causes chronic gastrointestinal problems in newborn babies, due to defects in a protein that transports various cellular components. Because they cannot properly absorb nutrition from food, these tiny patients require intravenous feeding almost immediately, which carries a high risk for sepsis and intestinal injury.
Engevik and her team study this disease using a mouse model that replicates many of the characteristics of the disorder in humans [1]. Interestingly, when Engevik gets together with her family, she isn’t the only one talking about MVID and villi. Her two sisters, Mindy and Kristen, also study the basic science of gastrointestinal disorders! Instead of sibling rivalry, though, this close alliance has strengthened the quality of her research, says Amy, who is the middle child.
Beyond advancing science and nurturing sisterhood in science, Engevik’s work also captured the fancy of the judges for the Federation of American Societies for Experimental Biology’s annual BioArt Scientific Image and Video Competition. Her image was one of 10 winners announced in December 2020.
Because multiple models are useful for understanding fundamentals of diseases like MVID, Engevik has also developed a large-animal model (pig) that has many features of the human disease [2]. She hopes that her efforts will help to improve our understanding of MVID and other digestive diseases, as well as lead to new, potentially life-saving treatments for babies suffering from MVID.
References:
[1] Loss of MYO5B Leads to reductions in Na+ absorption with maintenance of CFTR-dependent Cl- secretion in enterocytes. Engevik AC, Kaji I, Engevik MA, Meyer AR, Weis VG, Goldstein A, Hess MW, Müller T, Koepsell H, Dudeja PK, Tyska M, Huber LA, Shub MD, Ameen N, Goldenring JR. Gastroenterology. 2018 Dec;155(6):1883-1897.e10.
[2] Editing myosin VB gene to create porcine model of microvillus inclusion disease, with microvillus-lined inclusions and alterations in sodium transporters. Engevik AC, Coutts AW, Kaji I, Rodriguez P, Ongaratto F, Saqui-Salces M, Medida RL, Meyer AR, Kolobova E, Engevik MA, Williams JA, Shub MD, Carlson DF, Melkamu T, Goldenring JR. Gastroenterology. 2020 Jun;158(8):2236-2249.e9.
Links:
Microvillus inclusion disease (Genetic and Rare Diseases Center/NIH)
Digestive Diseases (National Institute of Diabetes and Digestive and Kidney Diseases/NIH)
Amy Engevik (Medical University of South Carolina, Charleston)
Podcast: A Tale of Three Sisters featuring Drs. Mindy, Amy, and Kristen Engevik (The Immunology Podcast, April 29, 2021)
BioArt Scientific Image and Video Competition (Federation of American Societies for Experimental Biology, Bethesda, MD)
NIH Support: National Institute of Diabetes and Digestive and Kidney Diseases
Using Frogs to Tackle Kidney Problems
Posted on by Dr. Francis Collins

Many human cells are adorned with hair-like projections called cilia. Scientists now realize that these specialized structures play many important roles throughout the body, including directing or sensing various signals such as fluid flow. Their improper function has been linked to a wide range of health conditions, such as kidney disease, scoliosis, and obesity.
Studying cilia in people can be pretty challenging. It’s less tricky in a commonly used model organism: Xenopus laevis, or the African clawed frog. This image highlights a healthy patch of motile cilia (yellow) on embryonic skin cells (red) of Xenopus laevis. The cilia found in humans and all other vertebrates are built from essentially the same elongated structures known as microtubules. That’s why researchers can learn a lot about human cilia by studying frogs.
Gene Editing in Dogs Boosts Hope for Kids with Muscular Dystrophy
Posted on by Dr. Francis Collins

Caption: A CRISPR/cas9 gene editing-based treatment restored production of dystrophin proteins (green) in the diaphragm muscles of dogs with Duchenne muscular dystrophy.
Credit: UT Southwestern
CRISPR and other gene editing tools hold great promise for curing a wide range of devastating conditions caused by misspellings in DNA. Among the many looking to gene editing with hope are kids with Duchenne muscular dystrophy (DMD), an uncommon and tragically fatal genetic disease in which their muscles—including skeletal muscles, the heart, and the main muscle used for breathing—gradually become too weak to function. Such hopes were recently buoyed by a new study that showed infusion of the CRISPR/Cas9 gene editing system could halt disease progression in a dog model of DMD.
As seen in the micrographs above, NIH-funded researchers were able to use the CRISPR/Cas9 editing system to restore production of a critical protein, called dystrophin, by up to 92 percent in the muscle tissue of affected dogs. While more study is needed before clinical trials could begin in humans, this is very exciting news, especially when one considers that boosting dystrophin levels by as little as 15 percent may be enough to provide significant benefit for kids with DMD.
Snapshots of Life: An Elegant Design
Posted on by Dr. Francis Collins

Credit: David Sleboda and Thomas Roberts, Brown University, Providence, RI
Over the past few years, my blog has highlighted winners from the annual BioArt contest sponsored by the Federation of American Societies for Experimental Biology (FASEB). So, let’s keep a good thing going with one of the amazing scientific images that captured top honors in FASEB’s latest competition: a scanning electron micrograph of the hamstring muscle of a bullfrog.
That’s right, a bullfrog, For decades, researchers have used the American bullfrog, Rana catesbeiana, as a model for studying the physiology and biomechanics of skeletal muscles. My own early work with electron microscopy, as a student at Yale in the 1970s, was devoted to producing images from this very tissue. Thanks to its disproportionately large skeletal muscles, this common amphibian has played a critical role in helping to build the knowledge base for understanding how these muscles work in other organisms, including humans.
Revealed in this picture is the intricate matrix of connective tissue that holds together the frog’s hamstring muscle, with the muscle fibers themselves having been digested away with chemicals. And running diagonally, from lower left to upper right, you can see a band of fibrils made up of a key structural protein called collagen.
Cool Videos: Fireworks under a Microscope
Posted on by Dr. Francis Collins
This Fourth of July, many of you will spread out a blanket and enjoy an evening display of fireworks with their dramatic, colorful bursts. But here’s one pyrotechnic pattern that you’ve probably never seen. In this real-time video, researchers set off some fluorescent fireworks under their microscope lens while making an important basic discovery about how microtubules, the hollow filaments that act as the supportive skeleton of the cell, dynamically assemble during cell division.The video starts with a few individual microtubule filaments (red) growing linearly at one end (green). Notice the green “comets” that quickly appear, followed by a red trail. Those are new microtubules branching off. This continuous branching is interesting because microtubules were generally thought to grow linearly in animal cells (although branching had been observed a few years earlier in fission yeast and plant cells). The researchers, led by Sabine Petry, now at Princeton University, Princeton, NJ, showed for the first time that not only do new microtubules branch during cell division, but they do so very rapidly, going from a few branches to hundreds in a matter of minutes [1].
Ferreting Out Genomic Secrets
Posted on by Dr. Francis Collins
Not only is the ferret (Mustela putorius furo) adept at navigating a dirt field or threading electrical cables through piping (in New Zealand, ferrets can be registered as electrician assistants), this furry 5-pounder ranks as a real heavyweight for studying respiratory diseases. In fact, much of our current thinking about influenza is influenced by research with ferrets.
Now, the ferret will stand out even more. As reported online in Nature Biotechnology, NIH-funded researchers recently sequenced the genome of the sable ferret, the type that is bred in the United States as a pet. By studying this genetic blueprint like an explorer would a map, scientists can perform experiments to learn more systematically how the ferret copes biologically with common or emerging respiratory pathogens, pointing the way to improved strategies to preserve the health and well being of humans and ferrets alike.
Drug Discovery from A to Z … Arrhythmias to Zebrafish!
Posted on by Dr. Francis Collins
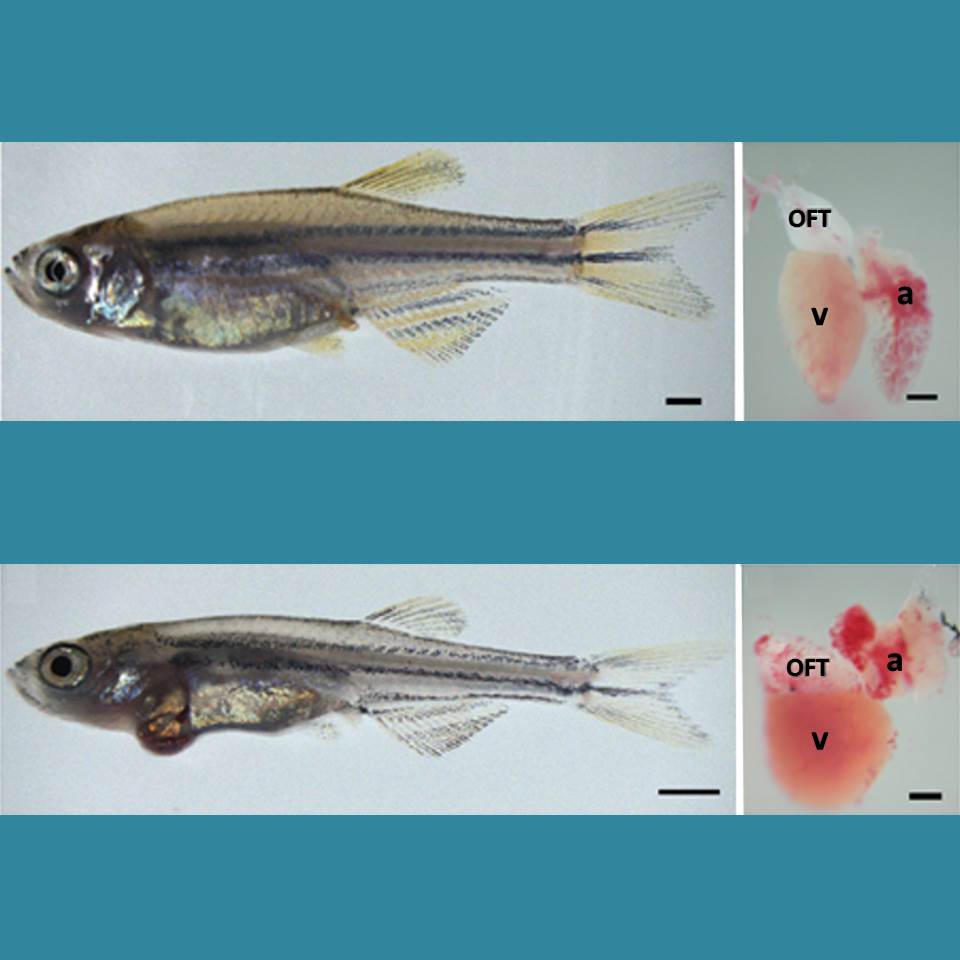
Caption: Healthy zebrafish (top) compared to zebrafish with arrhythmia-causing mutation (bottom). Their hearts are shown to the right, with enlargement indicating a weaker heart. The heart’s outflow tract is marked OFT; atrium, a; and ventricle, v.
Credit: Asimaki et al. Science Translational Medicine
Arrhythmia is a condition in which the heart loses its regular rhythm, beating either too rapidly or too slowly. Occasional irregular heartbeats are harmless, but if sustained they can cause dizziness, fainting, and even sudden death. There are a number of drugs available that can prevent arrhythmias, but none are perfect. Implanted devices can help—pacemakers can keep the heart from beating too slowly, and defibrillators can reset the heart’s rhythm with an electrical shock if a dangerously rapid rhythm develops.
But new treatments are needed. Now, an NIH-funded research team has created an animal model that is advancing efforts to find new drugs to prevent arrhythmia. Led by Jeffrey Saffitz at Beth Israel Deaconess Medical Center, Boston, researchers used genetic engineering techniques to produce zebrafish with genetic mutations identical to those in some people who suffer from a rare inherited disease called arrhythmogenic cardiomyopathy (ACM). In humans, ACM leads to dangerous arrhythmias that can cause sudden cardiac death, usually in people under the age of 35.
Of Mice, Men, and Medicine
Posted on by Dr. Francis Collins

Source: Wyss Institute and Bill Branson, NIH
The humble laboratory mouse has taught us a phenomenal amount about embryonic development, disease, and evolution. And, for decades, the pharmaceutical industry has relied on these critters to test the safety and efficacy of new drug candidates. If it works in mice, so we thought, it should work in humans. But when it comes to molecules designed to target a sepsis-like condition, 150 drugs that successfully treated this condition in mice later failed in human clinical trials—a heartbreaking loss of decades of research and billions of dollars. A new NIH-funded study [1] reveals why.