mouse model
DNA Base Editing May Treat Progeria, Study in Mice Shows
Posted on by Dr. Francis Collins
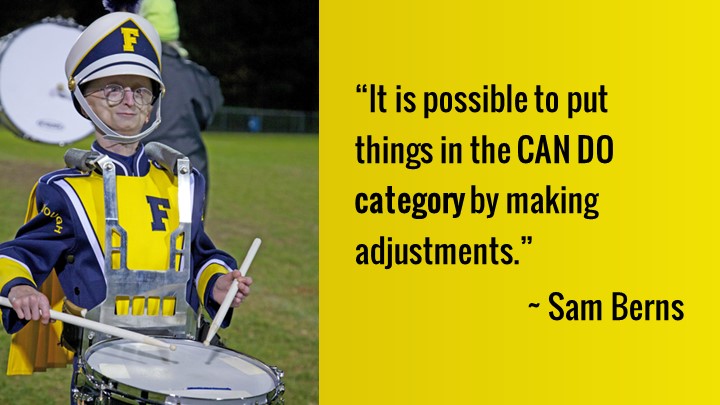
My good friend Sam Berns was born with a rare genetic condition that causes rapid premature aging. Though Sam passed away in his teens from complications of this condition, called Hutchinson-Gilford progeria syndrome, he’s remembered today for his truly positive outlook on life. Sam expressed it, in part, by his willingness to make adjustments that allowed him, in his words, to put things that he always wanted to do in the “can do” category.
In this same spirit on behalf of the several hundred kids worldwide with progeria and their families, a research collaboration, including my NIH lab, has now achieved a key technical advance to move non-heritable gene editing another step closer to the “can do” category to treat progeria. As published in the journal Nature, our team took advantage of new gene-editing tools to correct for the first time a single genetic misspelling responsible for progeria in a mouse model, with dramatically beneficial effects [1, 2]. This work also has implications for correcting similar single-base typos that cause other inherited genetic disorders.
The outcome of this work is incredibly gratifying for me. In 2003, my NIH lab discovered the DNA mutation that causes progeria. One seemingly small glitch—swapping a “T” in place of a “C” in a gene called lamin A (LMNA)—leads to the production of a toxic protein now known as progerin. Without treatment, children with progeria develop normally intellectually but age at an exceedingly rapid pace, usually dying prematurely from heart attacks or strokes in their early teens.
The discovery raised the possibility that correcting this single-letter typo might one day help or even cure children with progeria. But back then, we lacked the needed tools to edit DNA safely and precisely. To be honest, I didn’t think that would be possible in my lifetime. Now, thanks to advances in basic genomic research, including work that led to the 2020 Nobel Prize in Chemistry, that’s changed. In fact, there’s been substantial progress toward using gene-editing technologies, such as the CRISPR editing system, for treating or even curing a wide range of devastating genetic conditions, such as sickle cell disease and muscular dystrophy
It turns out that the original CRISPR system, as powerful as it is, works better at knocking out genes than correcting them. That’s what makes some more recently developed DNA editing agents and approaches so important. One of them, which was developed by David R. Liu, Broad Institute of MIT and Harvard, Cambridge, MA, and his lab members, is key to these latest findings on progeria, reported by a team including my lab in NIH’s National Human Genome Research Institute and Jonathan Brown, Vanderbilt University Medical Center, Nashville, TN.
The relatively new gene-editing system moves beyond knock-outs to knock-ins [3,4]. Here’s how it works: Instead of cutting DNA as CRISPR does, base editors directly convert one DNA letter to another by enzymatically changing one DNA base to become a different base. The result is much like the find-and-replace function used to fix a typo in a word processor. What’s more, the gene editor does this without cutting the DNA.
Our three labs (Liu, Brown, and Collins) first teamed up with the Progeria Research Foundation, Peabody, MA, to obtain skin cells from kids with progeria. In lab studies, we found that base editors, targeted by an appropriate RNA guide, could successfully correct the LMNA gene in those connective tissue cells. The treatment converted the mutation back to the normal gene sequence in an impressive 90 percent of the cells.
But would it work in a living animal? To get the answer, we delivered a single injection of the DNA-editing apparatus into nearly a dozen mice either three or 14 days after birth, which corresponds in maturation level roughly to a 1-year-old or 5-year-old human. To ensure the findings in mice would be as relevant as possible to a future treatment for use in humans, we took advantage of a mouse model of progeria developed in my NIH lab in which the mice carry two copies of the human LMNA gene variant that causes the condition. Those mice develop nearly all of the features of the human illness
In the live mice, the base-editing treatment successfully edited in the gene’s healthy DNA sequence in 20 to 60 percent of cells across many organs. Many cell types maintained the corrected DNA sequence for at least six months—in fact, the most vulnerable cells in large arteries actually showed an almost 100 percent correction at 6 months, apparently because the corrected cells had compensated for the uncorrected cells that had died out. What’s more, the lifespan of the treated animals increased from seven to almost 18 months. In healthy mice, that’s approximately the beginning of old age.
This is the second notable advance in therapeutics for progeria in just three months. Last November, based on preclinical work from my lab and clinical trials conducted by the Progeria Research Foundation in Boston, the Food and Drug Administration (FDA) approved the first treatment for the condition. It is a drug called Zokinvy, and works by reducing the accumulation of progerin [5]. With long-term treatment, the drug is capable of extending the life of kids with progeria by 2.5 years and sometimes more. But it is not a cure.
We are hopeful this gene editing work might eventually lead to a cure for progeria. But mice certainly aren’t humans, and there are still important steps that need to be completed before such a gene-editing treatment could be tried safely in people. In the meantime, base editors and other gene editing approaches keep getting better—with potential application to thousands of genetic diseases where we know the exact gene misspelling. As we look ahead to 2021, the dream envisioned all those years ago about fixing the tiny DNA typo responsible for progeria is now within our grasp and getting closer to landing in the “can do” category.
References:
[1] In vivo base editing rescues Hutchinson-Gilford Progeria Syndrome in mice. Koblan LW et al. Nature. 2021 Jan 6.
[2] Base editor repairs mutation found in the premature-ageing syndrome progeria. Vermeij WP, Hoeijmakers JHJ. Nature. 6 Jan 2021.
[3] Programmable editing of a target base in genomic DNA without double-stranded DNA cleavage. Komor AC, Kim YB, Packer MS, Zuris JA, Liu DR. Nature. 2016 May 19;533(7603):420-424.
[4] Programmable base editing of A•T to G•C in genomic DNA without DNA cleavage. Gaudelli NM, Komor AC, Rees HA, Packer MS, Badran AH, Bryson DI, Liu DR. Nature. 2017 Nov 23;551(7681):464-471.
[5] FDA approves first treatment for Hutchinson-Gilford progeria syndrome and some progeroid laminopathies. Food and Drug Administration. 2020 Nov 20.
Links:
Progeria (Genetic and Rare Diseases Information Center/NIH)
What are Genome Editing and CRISPR-Cas9? (National Library of Medicine/NIH)
Somatic Cell Genome Editing Program (Common Fund/NIH)
David R. Liu (Harvard University, Cambridge, MA)
Collins Group (National Human Genome Research Institute/NIH)
Jonathan Brown (Vanderbilt University Medical Center, Nashville, TN)
NIH Support: National Human Genome Research Institute; National Center for Advancing Translational Sciences; National Institute of Biomedical Imaging and Bioengineering; National Institute of Allergy and Infectious Diseases; National Institute of General Medical Sciences; Common Fund
Study Suggests Repurposed Drugs Might Treat Aggressive Lung Cancer
Posted on by Dr. Francis Collins

Credit: Leanne Li, Koch Institute at MIT
Despite continued progress in treatment and prevention, lung cancer remains our nation’s leading cause of cancer death. In fact, more Americans die of lung cancer each year than of breast, colon, and prostate cancers combined [1,2]. While cigarette smoking is a major cause, lung cancer also occurs in non-smokers. I’m pleased to report discovery of what we hope will be a much-needed drug target for a highly aggressive, difficult-to-treat form of the disease, called small cell lung cancer (SCLC).
Using gene-editing technology to conduct a systematic, large-scale search for druggable vulnerabilities in certain types of cancer cells grown in lab dishes, NIH-funded researchers recently identified a metabolic pathway that appears to play a key role in SCLC. What makes this news even more encouraging is drugs that block this pathway already exist. That includes one in clinical testing for other types of cancer, and another that’s FDA-approved and has been safely used for more than 20 years to treat people with rheumatoid arthritis.
The new work comes from the lab of Tyler Jacks, Massachusetts Institute of Technology (MIT), Cambridge. The Jacks lab, which is dedicated to understanding the genetic events that lead to cancer, develops mouse models engineered to carry the same genetic mutations that turn up in human cancers.
In work described in Science Translational Medicine, the team, co-led by Leanne Li and Sheng Rong Ng, applied CRISPR gene-editing tools to cells grown from some of their mouse models. Aiming high in terms of scale, researchers used CRISPR to knock out systematically, one by one, each of about 5,000 genes in cells from the SCLC mouse model, as well in cells from mouse models of other types of lung and pancreatic cancers. They looked to see what gene knockouts would slow down or kill the cancer cells, because that would be a good indication that the protein products of these genes, or the pathways they mediated, would be potential drug targets.
Out of those thousands of genes, one rose to the top of the list. It encodes an enzyme called DHODH (dihydroorotate dehydrogenase). This enzyme plays an important role in synthesizing pyrimidine, which is a major building block in DNA and RNA. Cytosine and thymine, the C and T in the four-letter DNA code, are pyrimidines; so is uracil, the U in RNA that takes the place of T in DNA. Because cancer cells are constantly dividing, there is a continual need to synthesize new DNA and RNA molecules to support the production of new daughter cells. And that means, unlike healthy cells, cancer cells require a steady supply of pyrimidine.
It turns out that the SCLC cells have an unexpected weakness relative to other cancer cells: they don’t produce as much pyrimidine. As a result, the researchers found blocking DHODH left the cells short on pyrimidine, leading to reduced growth and survival of the cancer.
This was especially good news because DHODH-blocking drugs, including one called brequinar, have already been tested in clinical trials for other cancers. In fact, brequinar is now being explored as a potential treatment for acute myeloid leukemia.
Might brequinar also hold promise for treating SCLC? To explore further, the researchers looked again to their genetic mouse model of SCLC. Their studies showed that mice treated with brequinar lived about 40 days longer than control animals. That’s a significant survival benefit in this system.
Brequinar treatment appeared to work even better when combined with other approved cancer drugs in mice that had SCLC cells transplanted into them. Further study in mice carrying SCLC tumors derived from four human patients added to this evidence. Two of the four human tumors shrunk in mice treated with brequinar.
Of course, mice are not people. But the findings suggest that brequinar or another DHODH blocker might hold promise as a new way to treat SCLC. While more study is needed to understand even better how brequinar works and explore potentially promising drug combinations, the fact that this drug is already in human testing for another indication suggests that a clinical trial to explore its use for SCLC might happen more quickly.
More broadly, the new findings show the promise of gene-editing technology as a research tool for uncovering elusive cancer targets. Such hard-fought discoveries will help to advance precise approaches to the treatment of even the most aggressive cancer types. And that should come as encouraging news to all those who are hoping to find new answers for hard-to-treat cancers.
References:
[1] Cancer Stat Facts: Lung and Bronchus Cancer (National Cancer Institute/NIH)
[2] Key Statistics for Lung Cancer (American Cancer Society)
[3] Identification of DHODH as a therapeutic target in small cell lung cancer. Li L, Ng SR, Colón CI, Drapkin BJ, Hsu PP, Li Z, Nabel CS, Lewis CA, Romero R, Mercer KL, Bhutkar A, Phat S, Myers DT, Muzumdar MD, Westcott PMK, Beytagh MC, Farago AF, Vander Heiden MG, Dyson NJ, Jacks T. Sci Transl Med. 2019 Nov 6;11(517).
Links:
Small Cell Lung Cancer Treatment (NCI/NIH)
Video: Introduction to Genome Editing Using CRISPR Cas9 (NIH)
Tyler Jacks (Massachusetts Institute of Technology, Cambridge)
NIH Support: National Cancer Institute
Creative Minds: Taking Aim at Adverse Drug Reactions
Posted on by Dr. Francis Collins

Sherrie Divito
As a practicing dermatologist, Sherrie Divito sees lots of patients each week at Brigham and Women’s Hospital, Boston. She also sees lots of research opportunities. One that grabbed her attention is graft-versus-host disease (GvHD), which can arise after a bone-marrow transplant for leukemia, lymphoma, or various other diseases. What happens is immune cells in the donated marrow recognize a transplant patient’s body as “foreign” and launch an attack. Skin is often attacked first, producing a severe rash that is a harbinger of complications to come in other parts of the body.
But Divito saw something else: it’s virtually impossible to distinguish between an acute GvHD-caused rash and a severe skin reaction to drugs, from amoxicillin to carbamazepine. In her GvHD studies, Divito had been researching a recently identified class of immune cell called tissue-resident memory T (Trm) cells. They remain in skin rather than circulating in the bloodstream. The clinical similarities made Divito wonder whether Trm cells may also help to drive severe skin allergies to drugs.
Divito has received a 2016 NIH Director’s Early Independence Award to find out. If correct, Divito will help not only to improve the lives of thousands of people with GvHD, but potentially benefit the millions of other folks who experience adverse reactions to drug.
DNA and the Roots of Hair Roots
Posted on by Dr. Francis Collins

Source: National Cancer Institute, NIH; Bill Branson, photographer.
It’s intriguing to find the roots of physical traits: skin color, height, and those weird tufts of hair on Uncle Mike’s ears. We’re all curious to know why we look the way we do. But new technologies are allowing us to discover the precise genetic roots of human traits that vary across the world. Variations in our DNA have helped us resist diseases and adapt to different climates and foods, enabling us to colonize just about every environment on the planet.
Recent studies have pinpointed variations responsible for lighter skin in Northern climates (such as SLC24A5 [1]) and the ability to tolerate milk sugar (lactose) in adulthood [2]. But a new NIH-funded study of a gene variant that arose in China adds a fascinating wrinkle—the use of a mouse model to help understand a potential human advantage [3]. (Regular readers will note that last week in this space I wrote about how mouse models could sometimes be misleading—this week the mouse is a champion!)